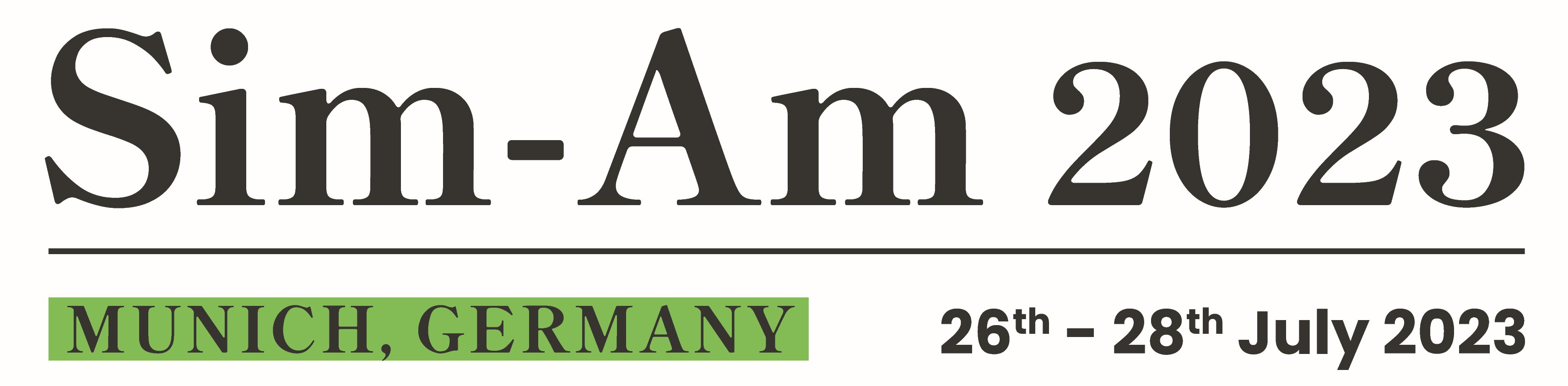
A Highly Efficient Computational Model for Fast Scan-Resolved Simulations of Metal Additive Manufacturing Processes on the Scale of Real Parts
Please login to view abstract download link
We present a fast method to simulate metal powder bed fusion additive manufacturing (PBFAM) processes on the part-scale in order to predict quantities of interest (QOI) such as temperature evolution, thermally induced dimensional warping and residual stresses. The fundamental computational challenge for part-scale simulations lies not so much in the requirements of the spatial approximation but rather in the large number of time steps necessary to resolve the fast moving laser path. While most models for part-scale simulation in the literature circumvent this problem by the use of homogenized, layerwise heat sources (sometimes applied to several physical layers at once), our model resolves the actual scan path. This enables new and detailed insights into the scan-strategy-dependent evolution of QOIs, especially for complex geometries. The unprecedented performance of the proposed thermal model is reached by a combination of different numerical and implementation techniques. The time step necessary to capture the moving heat source is close to the critical time step of an explicit Euler time integration scheme. Thus, explicit time integration is chosen for the active laser phase, and implicit schemes (which allows for large time steps) are chosen for the interlayer cool down phase. By the use of a lumped thermal capacity, the explicit time integration scheme does not require the solution of a linear system of equations, and the time stepping becomes a straight-forward update procedure. Thus, the wall time per computed time step is determined by the speed with which the explicit update rule can be evaluated. We use well-established techniques for parallel evaluation on distributed, adaptively-refined meshes to distribute the work among available CPUs. To utilize the capabilities of a modern CPU, the implementation uses vectorization over cells, which allows to perform the same computation for multiple data at once. All vector accesses and updates are performed in a cache-efficient manner. In summary, the combination of the aforementioned modeling choices and implementation techniques leads to a wall time per computational step in the millisecond range as long as a sufficient number of CPUs is employed. This enables us perform thermal simulations with consistently resolved laser beam path for the complete build process of the AM Bench 2022 cantilever specimen (312 layers, ~10 million spatial DoFs, ~50 million time steps) with a time to solution below one day.